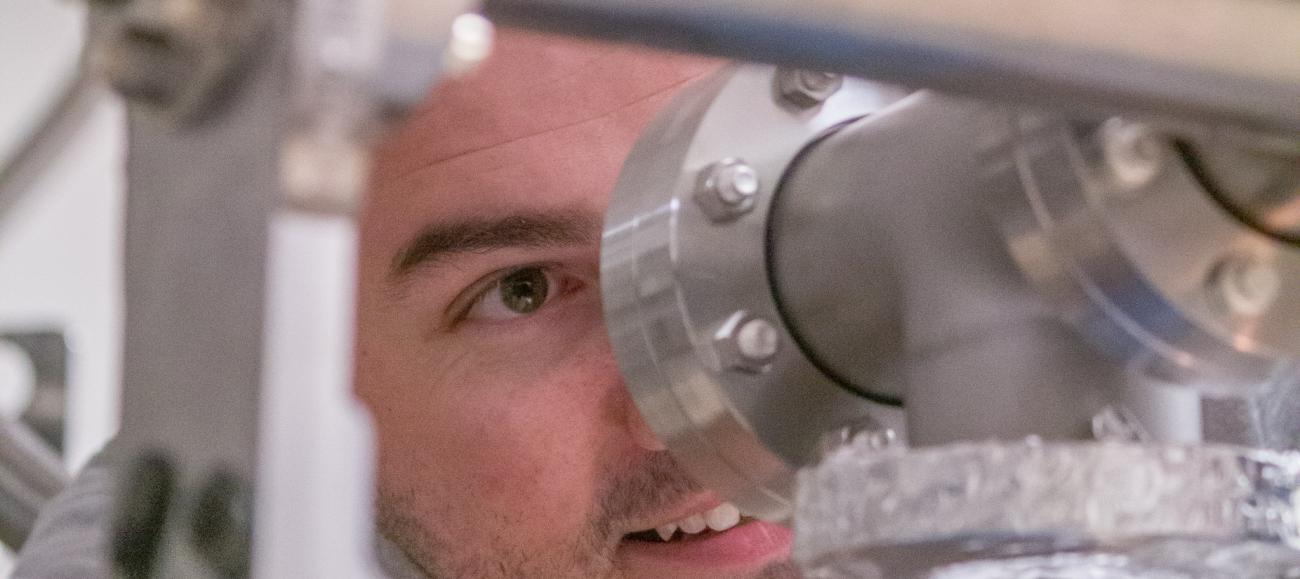
PhD Research Rotation
First year Department of Physics PhD students may use this form to select their research rotation preferences.
*Students must sign-in to view form.
Research Rotations
Faculty Name | Type | Research Area | Research Title | Research/Project Short Description |
---|---|---|---|---|
Andrei Belitsky | Theoretical | Cosmology, Particle, and Astrophysics | Scattering amplitudes in gauge theories and Feynman integrals | The sector of the Standard Model of Particle Physics dealing with strong interactions is described by Quantum Chromodynamics. The strong force between elementary constituents of matter is responsible for its very existence. However, to date, analytical treatment of the strong interaction is beyond the grasp of analytic field-theoretical methods. Thus, the bulk of applications is based on factorization theorems which separate the physics of short and long distances. The short-distance portion is calculable in terms of scattering of elementary constituents of matter (quarks and gluons). Quantum effect are of paramount importance for achieving theoretical accuracy on par with experimental uncertainties. This requires calculation of multiloop Feynman integrals. The latter are amenable to a number of field-theoretical techniques. The current project is intended to introduce a graduate student to this subject. |
Antia S. Botana | Theoretical | Nanoscience and Materials Physics | Computational design of novel superconducting materials | Superconductivity is a quantum state of matter whereby electrons move through a material without resistance. Superconducting materials are of paramount importance to different technologies: from magnetic resonance imaging machines to accelerators for high-energy physics, and magnetic levitating trains. However, their usefulness is often restricted by the prohibitively low temperatures at which superconductivity usually emerges. Increasing this transition temperature and discovering new superconductors has been limited by the lack of consensus as to what causes superconductivity in some classes of materials. One of these classes is represented by copper oxides whose discovery in the 1980s had a profound influence on physics as they could superconduct at a higher temperature than any material known at the time. This project will use advanced computational and theoretical methods to investigate nickel and palladium oxides - promising candidate materials for superconductivity based on the proximity of nickel and palladium to copper in the periodic table. The goal of the project is to discover a new family of superconductors while providing insights into the origin of high-temperature superconductivity. You will develop computational approaches suited for superconducting materials and perform simulations to establish the differences and similarities between the physics of nickel, palladium, and copper oxides. This project can be continued as your PhD topic. See some of our recent publications on this topic on our webpage or Google Scholar (Antia S. Botana). |
Antia S. Botana | Theoretical | Nanoscience and Materials Physics | Discovery and Control of Skyrmions in 2D van der Waals Magnets | Magnetic skyrmions are topologically protected spin textures with exciting quantum properties towards information and neuro-inspired technologies. To date, the experimentally known skyrmionic platforms are restricted to bulk crystals and metallic multilayer films. The ultimate goal of this project is to realize skyrmions in two-dimensional (2D) and van der Waals layered materials and investigate emergent properties arising from their reduced dimensions. The project will use advanced computational methods (from density functional theory to Monte-Carlo) to study transition metal dihalide monolayers and their moiré superlattices as a platform for skyrmion realization. The goal of the project is to establish what hallmark characteristics are important for skyrmion formation in the monolayer and few-layer limit. You will be able to explore different mechanisms for skyrmion realization using computational techniques to achieve this goal: from inversion symmetry breaking in ferromagnetic monolayers to twisting. This project can be continued as your PhD topic. See some of our recent publications on this topic on our webpage or Google Scholar (Antia S. Botana). |
Arunima Singh | Theoretical | Nanoscience and Materials Physics | Ab-Initio Theory and Simulations of 2D Materials | This project will involve the study of 2D materials' heterostructures for nanoelectronics and solar-energy conversion applications. You will be using density-functional theory simulations, excited state theory simulations, and machine learning methods to study the fundamental interface structure, charge transfer at the interface, band offsets, and molecular affinity of the heterostructures. The work done in this rotation will be extendable to your thesis. |
Arunima Singh | Theoretical | Nanoscience and Materials Physics | Excited State Theory Simulations of Ultra Materials | In this project, you will be studying the charged defects in ultra-wideband gap (UWBG) materials via excited state theory simulations. The simulations will be performed under the GW approximation and will involve studying the charge-transition levels in UWBG material AlN, which is widely used for power electronics. The work done in this rotation will be extendable to your thesis. For more information about our research group see https://cmd.lab.asu.edu/ |
Banu Ozkan | Theoretical | Biological and Soft Matter Physics | The thermodynamic paradox of dorman state in bacteria | Bacterial spores represent the most dormant and resilient state of any known viable cell. Spores are found everywhere on our planet, from the oceans to the desert, from the tropics to the arctic and even in the stratosphere. However, wild-type isolates of bacterial spores remain stable over many years, even when stored in water, and do not exhibit spontaneous germination (i.e. going back to the active/live state). The absence of spontaneous germination indicates that spores must reside in a deep free energy minimum near equilibrium. Such a minimum energy state can account for the absence of spontaneous germination, but poses yet another conundrum: How can spores germinate within minutes? The spore is not known to produce metabolic energy that would help it overcome a high free energy barrier and force its way towards a return to life. Furthermore, even if the spore can escape its deep dormant state, it is unclear how the spore can resume full physiological activity and start to grow and replicate so quickly. Specifically, how can a spore with no measurable physiological activity initiate all the various processes necessary for vegetative growth and replication in just a few minutes? Our objective is to resolve this paradox by developing a theoretical and mathematical framework based on nonequilibrium statistical mechanics and test its predictions directly through quantitative experiments. Successful outcome will provide a new and deep understanding of how a living cell can reside in an extremely dormant and stable state and yet retain the ability to rapidly return to life. This mission will lay the groundwork to develop novel methods to exert unprecedented control over spores and even take advantage of their unique biology. |
Banu Ozkan | Theoretical | Biological and Soft Matter Physics | Developing a novel framework to understanding long-range regulations conducted by ionic interactions in cell | The goal is to develop here a new mathematical framework based on a new hypothesis and biological concept, which we refer to as IonicAllostery. The effect of ligand binding at one site regulates the activity of a functional site at a distance. Monod considered his contribution to allostery even more significant than his Nobel Prize, claiming that he had discovered “the second secret of life”. We postulate that the concept of allostery goes beyond remote modulation within a protein and can couple distant processes within the cell. By pursuing our postulate of IonicAllostery, we aim to unravel life’s third secret: How allosteric regulation can propagate through ionic interactions among distant biological macromolecules to modulate cell physiology and function. Specifically, we will integrate coarse grained molecular models with cellular-level models to determine whether competition for a limited and dynamic pool of inorganic metal ions can give rise to unexpected long-range interaction between charged macromolecules, such as DNA, RNA and proteins. This work can thus reveal whether the “central dogma of biology” is subject to an unknown layer of control that couples fundamental processes such as transcription and translation through ionic interactions. |
Damien Easson | Theoretical | Cosmology, Particle, and Astrophysics | Exploration of non-canonical field theories in cosmological and gravitational systems. | Student will examine cosmological and black hole solutions in theories with non-standard kinetic terms. The goal is to discover novel applications of such theories to inflation, dark energy, bouncing cosmologies and/or compact objects such as black holes and wormholes. |
Damien Easson | Theoretical | Cosmology, Particle, and Astrophysics | Selected topics in high energy theoretical physics and gravity. | TBA |
dmitry matyushov | Theoretical | Biological and Soft Matter Physics | Calculation of diffusion coefficients of ions in water |
The project aims to calculate the effect of charge asymmetry on ionic mobility. Most proteins have charge distributed over their surfaces such that the center of mass and the center of charge do not coincide. Simulations of diffusion of proteins are computationally expensive and this project aims at a model study to understand the principles behind diffusion of particles with asymmetric charge distribution. A spherical solute will be placed in MD-Pol model of water and molecular dynamics simulation trajectories will be produced using LAMMPS. Those will be analyzed to calculate the diffusion coefficient by different algorithms to test the consistency. The simulations will be repeated with the center of charge shifted relative to the center of mass and the dependence of the diffusion coefficient on the shift magnitude will be produced. Basic knowledge of programming with Python or Mathematica will be required to analyze the data. |
Doug Shepherd | Experimental | Biological and Soft Matter Physics, Nanoscience and Materials Physics | New approaches to high-speed optical microscopy | One area of research of the Quantitative Imaging and Inference lab (QI2lab) is to push the boundaries of speed and resolution in optical microscopy. We recently demonstrated the first quantitative imaging of the microscale correlated Brownian motion for helices using light-sheet fluorescence microscopy and are now working towards perform optical diffraction tomography, as type of optical quantitative phase imaging, at 3D rates faster than 1,000 times per second. The goal of these microscopy and associated computational approaches are provide new insights into how biological systems interact with the laws of physics, such as the motion of individual viruses in the nucleus of a cell or how bacteria swimming different in bulk fluid versus near a solid surface. These methods can also be used for nanoscience, such as mapping charge transfer between adjacent semiconductor nanocrystals or density changes in atomic gases. The student will help prototype new optical microscopy approaches, work on the theory of light interacting with matter to improve tomography reconstruction, and perform experiments along other lab members. Skills learned can include laser alignment, calibration, instrument control electronics and programming, inverse modeling of optical microscopes, and quantitative modeling of soft matter or condensed matter systems. This project can be continued towards a PhD dissertation in Physics. |
Doug Shepherd | Experimental | Biological and Soft Matter Physics, Nanoscience and Materials Physics | New approaches to high-speed optical microscopy | One area of research of the Quantitative Imaging and Inference lab (QI2lab) is to push the boundaries of speed and resolution in optical microscopy. We recently demonstrated the first quantitative imaging of the microscale correlated Brownian motion for helices using light-sheet fluorescence microscopy and are now working towards perform optical diffraction tomography, as type of optical quantitative phase imaging, at 3D rates faster than 1,000 times per second. The goal of these microscopy and associated computational approaches are provide new insights into how biological systems interact with the laws of physics, such as the motion of individual viruses in the nucleus of a cell or how bacteria swimming different in bulk fluid versus near a solid surface. These methods can also be used for nanoscience, such as mapping charge transfer between adjacent semiconductor nanocrystals or density changes in atomic gases. The student will help prototype new optical microscopy approaches, work on the theory of light interacting with matter to improve tomography reconstruction, and perform experiments along other lab members. Skills learned can include laser alignment, calibration, instrument control electronics and programming, inverse modeling of optical microscopes, and quantitative modeling of soft matter or condensed matter systems. This project can be continued towards a PhD dissertation in Physics. |
Igor Shovkovy | Theoretical | Cosmology, Particle, and Astrophysics | Professor | Relativistic plasma under extreme conditions (strong magnetic fields, high temperatures), physics of heavy-ion collisions, neutron stars |
Igor Shovkovy | Theoretical | Cosmology, Particle, and Astrophysics | Chiral anomalous processes in magnetospheres of magnetars | Quantum field theoretical studies of chiral anomalous processes and their consequences in the gap regions of the magnetospheres of magnetars. |
Jingyue Liu | Experimental | Nanoscience and Materials Physics | Electrochemical Conversion of Molecules for Sustainable Energy | Design and develop nanoscale architectures for electrochemical conversion of molecules with a focus on producing clean energy; understand the fundamental processes of fabricating functional structures at the single-atom limit; and correlate synthesis-structure-performance relationships. |
Jose Menendez | Experimental | Nanoscience and Materials Physics | Spectroscopic ellipsometry and Raman scattering of semiconductors | The student will learn to perform spectroscopic ellipsometry measurements in the infrared and visible/UV as well as Raman scattering measurements. Ellipsometry is an optical technique that yields the complex dielectric function of the material. It is used to study the electronic structure and the effect of extrinsic perturbations such as doping. While ellipsometry looks at elastically reflected light, Raman spectroscopy investigates inelastically scattered light associated with elementary excitations in the sample, such as phonons. These are low-probability events that require laser excitation for their observation. Samples of current interest include GeSn alloys with very narrow band gaps that may have applications in the mid-IR. The analysis of Raman data provides important information on sample composition and strain, while the study of ellipsometry data provides critical clues on the electronic structure of the materials. In both cases, computer simulations are needed to extract the desired physical information, and the student will be trained in performing this analysis. This is a project for a student who is seriously considering a career in experimental physics. A significant advantage is that the skills the student will acquire are highly sought by potential employers in industry, so that the project will make a positive impact on the student’s CV even if he/she decides to complete a dissertation in some other field. |
Jose Menendez | Theoretical | Nanoscience and Materials Physics | Trap-assisted tunneling in semiconductor diodes | Trap-assisted tunneling is an important process that leads to electron-hole recombination in semiconductor diodes. While the area is very broad and requires a very strong background in semiconductor and device physics, this project focuses on a narrow (but important) technical point that can be handled during a research rotation. In semiconductors, traps act as intermediate states in the transfer of electrons from the conduction band to the valence band. Under the large electric fields that usually prevails in the depletion layers of devices, carriers can tunnel off the traps by emitting phonons or other elementary excitations. The process is quite complex and requires very elaborate quantum-mechanical calculations which are not suitable for device simulators. For this reason, researchers have developed approximate models that provide analytical expressions for the tunneling process. One of the most popular ones in a model by Hurkx, which reduces the problem to the calculation of a one-dimensional integral. This integral is itself approximated by an analytical expression, but under certain experimental conditions this approximation is rather poor. The purpose of this rotation is to test a new approximation of the Hurkx integral and to explore possible further improvements. This project will give the student the opportunity to familiarize themselves with the basic aspects of semiconductor device physics while making a contribution to the field that might be publishable. |
Jose Menendez | Theoretical | Nanoscience and Materials Physics | Semiconductor Equations using Polylogarithms | The purpose of this research is to develop an approach to the solution of the coupled semiconductor equations that transitions smoothly from the Boltzmann to the Fermi Dirac limit and is capable of simulating the operation of semiconductor devices at room and cryogenic temperatures. The approach must be compatible with current algorithms for the numerical solution of the equations. |
Kevin Schmidt | Theoretical | Cosmology, Particle, and Astrophysics, Nanoscience and Materials Physics | Quantum Monte Carlo Studies | I have several projects using quantum Monte Carlo methods to study quantum many-particle systems. These include novel superfluidity, cold atomic gases, and effective field theories of nucleons and mesons. These calculations generally require translation of theory into numerical computer programs, so programming ability or a willingness to learn to write computer programs is necessary. |
Kevin Schmidt | Theoretical | Cosmology, Particle, and Astrophysics, Nanoscience and Materials Physics | Quantum Monte Carlo Studies | I have several projects using quantum Monte Carlo methods to study quantum many-particle systems. These include novel superfluidity, cold atomic gases, and effective field theories of nucleons and mesons. These calculations generally require translation of theory into numerical computer programs, so programming ability or a willingness to learn to write computer programs is necessary. |
Maxim Sukharev | Theoretical | Nanoscience and Materials Physics | Electrodynamics at complex nanoscale interfaces: multiscale modeling and applications |
The research program described below is continuously funded through Air Force Office of Scientific Research and the Office of Naval Research. There will be two/three RA positions available soon. Successful candidates must first go through the research rotation to demonstrate their commitment and skills. The scientific community has recently shown avid interest in the intriguing behavior of molecular systems interacting with spatially confined electromagnetic radiation within optical cavities. This interest stems from the promise that such interactions affect chemical dynamics and thus could be used in innovative applications in chemistry and materials science. While certain manifestations of these phenomena are well-understood, the effects of the cavity environment on chemical processes and transport mechanisms still present a vibrant frontier for research and discovery. The research program aims to venture beyond traditional observational studies and build a more robust bridge between empirical data and theoretical predictions. By doing so, it aspires to shed new light on the mysteries of polaritonic chemistry. To that end, the program integrates theoretical and computational approaches to develop quantitative multiscale numerical tools for polaritonic chemistry that go far beyond simple qualitative modeling. Theoretical models, despite their apparent simplicity, provide the conceptual mathematical framework that underpins our understanding of polaritonic phenomena. These models guide our exploration and help us make sense of the rich tapestry of interactions. Complementing these, computational studies offer a controlled environment where we can simulate complex systems under various conditions and systematically test theoretical predictions. The research program aspires to weave these threads together, integrating computational electrodynamics with the quantum dynamics of large molecular systems. This combined approach will be used to investigate collective optical effects in plasmonic cavities of diverse configurations. Notably, the research methodology offers some unique advantages. For instance, it allows for the direct examination of short pulses and direct access to various transients on differing time scales, including those associated with chemical dynamics. Moreover, the approach facilitates the exploration of strong coupling effects induced in chiral nano-cavities. In summary, the research initiative aims to harness the power of computational and theoretical approaches to drive forward our understanding of polaritonic chemistry, and in doing so, open new avenues for discovery and application in chemistry and materials science. Advancing multiscale modeling on a quantitative level improves our ability to utilize computers for a cost-efficient chemical/biological sensors design. Future applications are envisioned for chemistry control in pre-designed plasmonic systems, nanoscale light sources in mid-infrared for chemical sensing and detection, infrared single-photon detection, and infrared counter measures. |
Mouzhe Xie | Experimental | Biological and Soft Matter Physics, Nanoscience and Materials Physics | Experimental Quantum BioSensing (EQuBS) lab -- quantum metrology and high precision measurement using nitrogen-vacancy defects in diamond crystal | As a new lab, we always have cross-disciplinary research projects available to postdocs, graduates, and undergrads! Please refer to https://sites.google.com/view/equbs-lab/opportunities for the latest update. Please email Prof. Mouzhe Xie (mouzhe.xie@asu.edu) for inquiries and to discuss details. |
Mouzhe Xie | Experimental | Biological and Soft Matter Physics, Nanoscience and Materials Physics | Experimental Quantum BioSensing (EQuBS) lab -- quantum metrology and high precision measurement using nitrogen-vacancy defects in diamond crystal | As a new lab, we always have cross-disciplinary research projects available to postdocs, graduates, and undergrads! Please refer to https://sites.google.com/view/equbs-lab/opportunities for the latest update. Please email Prof. Mouzhe Xie (mouzhe.xie@asu.edu) for inquiries and to discuss details. |
Navish Wadhwa | Experimental | Biological and Soft Matter Physics | Single-molecule imaging of bacterial flagellar stator | Can we "see" single proteins in action? In this project, you will develop fluorescently labeled versions of a protein that is directly responsible for allowing bacteria to swim around. You will image this protein in living cells using total internal reflection microscopy. From these images, you will quantify the behavior of this protein, such as diffusion and binding rates, to understand how various stimuli from the environment affect its activity. You will them perform theoretical calculations and computational analyses to identify and enumerate its behavioral states. Findings from this work will assist in improving the understanding of how cells and molecular machines adapt to their environment and how bacteria might use this to perform intracellular signaling. This project combines state-of-the-art biophysics experiments with theoretical modeling and will be suitable for any physics graduate students who are curious about the working of the living world. This project can be continued towards a PhD dissertation in Physics. The work is funded by National Institutes of Health under the grant GM134124. |
Navish Wadhwa | Experimental | Biological and Soft Matter Physics | Going with the flow: how bacteria deal with fluid flows | In nature as well as in disease, pathogens such as bacteria experience significant flow rates from the environment. For instance, during urinary tract infections, bacteria often move against urinary flow to reach the upper urinary tract including kidneys, which leads to a more severe infection. Despite its medical and scientific importance, the interaction between swimming bacteria and flows remains poorly understood. In this project, you will combine microfluidics, quantitative microscopy, low Reynolds number hydrodynamics, and theoretical biophysics to ask a simple question: What happens when we subject bacteria to flows? There will be a significant opportunity to develop this project into a competitive grant application and to continue this work as part of a PhD dissertation. |
Navish Wadhwa | Experimental | Biological and Soft Matter Physics | Dealing with stress: how cells respond to mechanical stimuli | A major goal of the Wadhwa lab (www.wadhwalab.com) is to understand how living matter responds to mechanical stimuli from their environment. Projects in our lab use bacteria as a model to experimentally investigate the interactions with cells and their physical environment. This includes how cells move within their environment (Thrust 1: Motility) and how they sense mechanical signals from their environment (Thrust 2: Mechanosensing). We have several projects within the lab that fall under one of both of these thrusts. Main experimental approaches in the lab include quantitative microscopy, microfluidics, electrorotation, and other forms of electric, magnetic, or fluidic perturbations. Theoretical work typically involve approaches related to stochastic processes and statistical physics. We welcome inquiries from new graduate students who are interested in exploring biological physics. We are happy to give you a lab tour and an opportunity to look at swimming bacteria under the microscope. Email Dr. Wadhwa (navish.wadhwa@asu.edu) for further information or to schedule an informal chat. |
Navish Wadhwa | Experimental | Biological and Soft Matter Physics | Dynamics of mechano-adaptation in bacterial flagella | Bacterial flagella are one of the most intricate molecular machines found in nature. These helical propellers power bacterial motility by generating hydrodynamic thrust that pushes bacteria through aquatic environments. Flagella are driven from the base, by a rotary biological motor known as the bacterial flagellar motor. Made up of over 20 different kinds of proteins, the bacterial flagellar motor is a canonical example of a biological nanomachine. In nature, bacteria inevitably experience dramatically different mechanical environments, such as water, mucus, soil, and tissues. How does the flagellar motor cope with large variations in mechanical load? To study this question experimentally, we have adopted the technique of electrorotation, in which a high-frequency rotating electric field applies an external torque on individual motors in single bacterial cells. Electrorotation allows us to apply step changes in mechanical load on the motor and to precisely quantify its response. Previous results show that the motor adapts to changes in load by dynamically adjusting the number of torque-generating subunits in its assembly. In other words, it continuously rebuilds itself to always keep its output (torque) matched to the demand (load). Bacterial flagella have an automatic gearshift, just like our cars. This rotation project will pursue the cutting edge of the question of flagellar mechano-adaptation. You will be involved in building electrorotation apparatus, conducting experiments with bacteria, analyzing data, and writing the results. Other projects in the general area of bacterial biomechanics are also available. Contact Dr. Wadhwa for more details. |
Navish Wadhwa | Experimental | Biological and Soft Matter Physics | Osmosensing and osmoregulation in bacteria | In order to survive in their natural environments, bacteria must rapidly adapt and respond to a variety of chemical and physical stresses. While bacterial sensing and adaptation to chemicals is well-studied, how bacteria deal with mechanical stresses remains poorly explored. Osmotic stress is a common physical stress that bacterial routinely experience and it can lead to large deformations of the cell body even leading to cell death. Therefore, bacteria have evolved clever mechanisms to respond to osmotic perturbations quickly and efficiently. In this project, we will investigate how bacterial response to osmotic stress at high temporal resolution. We will apply osmotic perturbations to single bacteria in microfluidic cells and quantify their response using high-speed imaging. Combining these results with previously collected data, we aim to discover a previously unknown link between bacterial mechanics and cellular energetics. Other projects in the general area of bacterial biomechanics are also available. Contact Dr. Wadhwa for more details. |
Nicholas Rolston | Theoretical | Nanoscience and Materials Physics | Inorganic Metal Halide Perovskites for Direct Alphavoltaic Converters | One of the key drawbacks of photovoltaic devices is the intermittency of power generation based on limited solar radiation. Radioactive sources that involve alpha particle emitting isotopes have continuous decay processes with long half-life can provide a source of extended and reliable power generation if coupled with long-lasting materials/devices for alphavoltaics. Metal halide perovskites (MHPs) are a rising star in the next generation of photovoltaic materials with lab-reported power conversion efficiencies rivaling that of incumbent silicon technology after just over ten years of research. Furthermore, MHPs hold the potential for making high-performance, low-cost devices through solution-processing of Earth-abundant materials in polycrystalline phases compatible with in-line methods such as roll-to-roll processing. The bandgap is highly tunable based on replacing constituents of the ABX3 lattice structure, most notably through replacing the X-site with Br (or Cl) in place of I to increase the band gap over a range of nearly 2 eV. Although MHPs have been extensively studied in photovoltaic applications, they also have showed significant promise in radiation-based gamma detectors and space environments due to the inherent radiation hardness of the large atomic number constituents. MHPs have also been developed in fully inorganic phases using CsPbI3 and CsPbBr3 phases that exhibit superior thermal stability compared to the hybrid organic-inorganic counterparts. The Cs, Pb, and I/Br components are expected to be more stable under alpha (and other forms of) radiation compared to many of the alternative materials that have been used in direct alphavoltaic converters (DACs) to date. This project will involve solution-based processing of inorganic metal halide perovskites and measurement of structural, ionic, and mechanical changes along with interactions of radiation and operational conditions with associated structural and mechanical robustness of charge transport layers/electrodes and interfaces for DAC applications. |
Oliver Beckstein | Theoretical | Biological and Soft Matter Physics | Brute-force sampling of small molecule conformational landscapes | "Small molecules" describes the incredibly vast number of organic molecules with molecular weight < 1000 dalton that can regulate a biological process. Most pharmacological drugs are small molecules and hence understanding their behavior, such as how they interact with solvent or proteins, is crucial in drug development. Due to the presence of rotatable bonds, small molecules can adapt multiple distinct conformations but understanding which of these conformations are the most likely ones, i.e., the ones with the lowest free energy, is a difficult problem. In this project you will create a computational workflow to exhaustively enumerate small molecule conformers and then calculate their approximate free energy. You will then adapt or develop an algorithm to enumerate the minima in this high dimensional free energy landscape. In this project you will learn to apply statistical mechanics to molecular systems and to learn to write scientific code in the Python programming language. |
Onur Erten | Theoretical | Nanoscience and Materials Physics | Theory of bilayer quantum spin liquids | This project aims to study bilayers of spin-orbital extensions of Kitaev model. In particular, the research will involve building new models that preserve partial integrability and obtaining the phase diagrams via various methods. |
Onur Erten | Theoretical | Nanoscience and Materials Physics | Theory of topological Kondo insulators with long range interactions | This project will involve building exactly solvable interacting model of topological Kondo insulators with long range interactions. |
Philip Mauskopf | Experimental | Nanoscience and Materials Physics | Superconducting quantum devices | Work on a variety of superconducting devices including high frequency qubits, single photon detectors, microwave resonators and kinetic inductance detectors. |
Quan Qing | Experimental | Biological and Soft Matter Physics, Nanoscience and Materials Physics | Integration of Raman spectrometer with quantum conductance measurement for multi-parameter single-molecule identification | How do charges transport through a single molecule sandwiched between two metal electrodes? It has long been shown that the conductance strongly depends on the molecule’s structure, and the interface between the molecule and the metal surface. But such measurements have proved to be very delicate and conflicting results have been all over the map. For example, DNA molecules have been shown to be insulators, conductors as well as superconductors in different experiments. Indeed, with the rapidly expanding studies of conductivity of biomolecules, where hydrogen bonds, pi-pi stacking, hydrophobic interactions, and allosteric structures are actively involved, more and more results have been shown to fall outside the realm of conventional quantum tunneling theories. New developments at the intersect of physics and biology reveal a wide open field for exploration, promising new paradigms of how charges can transport in a 3D complex molecular systems, as well as label-free biosensors based on the dynamic conductance measurements. However, all studies have been significantly limited by the techniques for constructing the metal-molecule-metal structure, mostly based on scanning tunneling microscope, mechanical break junctions, or brute-force top-down lithography. All measurements so far rely on the random diffusion and binding of molecules, and statistical analysis of hundreds to thousands of repetitive operations. It is extremely challenging to control the position and states of the molecule bridge between electrodes, and the operation typically require strict environment control and with high technical skills. Is it possible to construct a platform for highly reproducible measurements that brings together single molecule delivery, conductivity measurements and molecule structure characterization, such that a broad range of biomolecules can be easily investigated under physiological conditions? The goal of this project is to develop a microchip-based universal platform that integrates nanopore, tunneling junction and Raman spectroscopy for multi-parameter investigation of different biomolecules in physiological conditions during enzymatic activities. The student will help the development of a customized Raman spectrometer and integrate with a nanoelectropore system to perform preliminary experiments on correlated Raman and quantum conductivity measurements on model molecules ranging from simple small molecules such as 4-mercaptobenzoic acid, to double-strand DNA molecules, and even larger proteins that can form molecule bridge through antibody-antigen interactions. |
Quan Qing | Experimental | Biological and Soft Matter Physics, Nanoscience and Materials Physics | Integration of Raman spectrometer with quantum conductance measurement for multi-parameter single-molecule identification | How do charges transport through a single molecule sandwiched between two metal electrodes? It has long been shown that the conductance strongly depends on the molecule’s structure, and the interface between the molecule and the metal surface. But such measurements have proved to be very delicate and conflicting results have been all over the map. For example, DNA molecules have been shown to be insulators, conductors as well as superconductors in different experiments. Indeed, with the rapidly expanding studies of conductivity of biomolecules, where hydrogen bonds, pi-pi stacking, hydrophobic interactions, and allosteric structures are actively involved, more and more results have been shown to fall outside the realm of conventional quantum tunneling theories. New developments at the intersect of physics and biology reveal a wide open field for exploration, promising new paradigms of how charges can transport in a 3D complex molecular systems, as well as label-free biosensors based on the dynamic conductance measurements. However, all studies have been significantly limited by the techniques for constructing the metal-molecule-metal structure, mostly based on scanning tunneling microscope, mechanical break junctions, or brute-force top-down lithography. All measurements so far rely on the random diffusion and binding of molecules, and statistical analysis of hundreds to thousands of repetitive operations. It is extremely challenging to control the position and states of the molecule bridge between electrodes, and the operation typically require strict environment control and with high technical skills. Is it possible to construct a platform for highly reproducible measurements that brings together single molecule delivery, conductivity measurements and molecule structure characterization, such that a broad range of biomolecules can be easily investigated under physiological conditions? The goal of this project is to develop a microchip-based universal platform that integrates nanopore, tunneling junction and Raman spectroscopy for multi-parameter investigation of different biomolecules in physiological conditions during enzymatic activities. The student will help the development of a customized Raman spectrometer and integrate with a nanoelectropore system to perform preliminary experiments on correlated Raman and quantum conductivity measurements on model molecules ranging from simple small molecules such as 4-mercaptobenzoic acid, to double-strand DNA molecules, and even larger proteins that can form molecule bridge through antibody-antigen interactions. |
Ricardo Alarcon | Experimental | Cosmology, Particle, and Astrophysics | Fundamental Physics Experiments with Cold and Ultra-cold Neutrons. | Work on different aspects connected with ongoing fundamental physics experiments: a precise measurement of the neutron beta decay and a search for the electric dipole moment of the neutron. |
Ricardo Alarcon | Experimental | Cosmology, Particle, and Astrophysics | The Electric Dipole Moment of the Neutron | We are presently working in mounting an experiment in Los Alamos National Laboratory searching for a non-zero electric dipole moment of the neutron (nEDM), whose possible existence is of fundamental interest and would significantly challenge theoretical extensions of the Standard Model (SM). The sensitivity reach of the nEDM experiment represents an improvement of about one order of magnitude with respect to the present sensitivity level. The discovery of a neutron EDM well above the SM value would be truly revolutionary and a significant challenge for theories of physics that go beyond the SM. This project is ideal for a graduate student looking for a Ph.D. thesis project. |
Ricardo Alarcon | Experimental | Physics and Society | Proton Computed Tomography for Cancer and Cardiac Arrhythmia Treatment | Proton CT (pCT) is widely recognized as a beneficial alternative to conventional X-ray CT for treatment planning in proton beam radiotherapy. In partnership with a small company, Proton Calibration Technologies (PCT) (www.protonct.com), and the Mayo Clinic Proton Center (https://www.mayoclinic.org/departments-centers/proton-beam-therapy-program/sections/overview/ovc-20185491) in Phoenix, AZ, we are embarking on a new project to develop pCT based on a novel algorithm developed by PCT. The development of a pCT system will follow the same general path that X-Ray CT used. We are looking for a new graduate student who would like to help us track this path to develop a pCT system. This will entail the design of instrumentation and data acquisition combined with sensitivity analysis via computer simulation. The Mayo Clinic Proton Center has agreed to provide beam time for these studies If you are interested, please get in touch with Professor Ricardo Alarcon (ralarcon@asu.edu). |
Richard Kirian | Experimental | Biological and Soft Matter Physics | Femtosecond x-ray biomolecular imaging technologies (various projects) | X-ray Free-Electron Lasers (XFELs) such as the Linac Coherent Light Source at SLAC National Lab produce extremely intense femtosecond pulses of x-rays. These pulses are sufficiently short in duration to detect motion on molecular timescales, and the wavelengths are sufficiently short to resolve molecular features at atomic resolution. Even though a single XFEL pulse can vaporize anything at its focus, the diffraction wavefront can "outrun" the destruction process and therefore allow for ultrafast, stroboscopic molecular imaging. In addition to the large (mile-long) XFEL facilities around the world (USA, Germany, Japan, Switzerland, South Korea), ASU is constructing the first compact femtosecond x-ray source that will have instruments dedicated to biomolecular imaging, which can provide a great opportunity for PhD student projects. There are numerous technologies under development in the Kirian lab for the purpose of imaging biomolecules with XFELs. These include algorithm and software development for the reconstruction of electron density maps from large diffraction datasets, and various types of "sample injection" hardware such as aerodynamically focused protein beams, electrodynamic traps, triggered liquid microdrops, high-speed nanojets, ultrathin sheet jets, 3D nanoprinted microfluidics, and so on. |
Richard Lebed | Theoretical | Cosmology, Particle, and Astrophysics | The Diabatic Dynamical Diquark Model | The period since 2003 has provided the first confirmed evidence for hadrons that do not fit into the known categories of quark-antiquark mesons or three-quark baryons. (An elementary knowledge of these particle categories and their basic features is a required starting point for the student.) Dozens of tetraquark and pentaquark states have so far been discovered. The internal structure of these states, however, remains a topic of theoretical debate, and several competing physical pictures have been proposed, each with its strengths and deficiencies. In the “dynamical diquark model”, a tetraquark consists of two bound quasiparticles: a two-quark diquark and a two-antiquark antidiquark. The purpose of this rotation is to become versed in the published literature of models for ordinary hadrons that are most closely related to the dynamical diquark model, namely, color flux-tube models and Born-Oppenheimer (BO) potentials that have been applied in the past to ordinary hadrons, and to learn how one can go beyond the BO approximation (“diabatic”) when more than one substructure is possible. Time permitting, the student will study the details of how the mixing of substructures in the diabatic model is simulated using code. |
Rizal Hariadi | Experimental | Biological and Soft Matter Physics, Nanoscience and Materials Physics | Chiral Metamaterials for Information Storage | Big data has increased the demand for technological innovations in data storage while traditional electronics are reaching their physical limit. DNA is an emerging data storage material as a viable alternative to silicon-based technology due to its high capacity and low operational energy. However, current DNA storage technologies lack the structural access and allocated organization of data encoded in DNA, and the core operations require complex and slow writing and readout processes such as sequencing, which leads to practical barriers in speed, sustainability, and scalability. To address these challenges, this project aims to develop the first DNA-encoded metamaterial data storage system, which could lead to a rewritable and high-speed integrated random access. Uniquely integrating DNA nanotechnology with an optical metamaterial platform, information bits will be designed and encoded by the helicity or handedness with DNA origami-enabled chiral meta-atoms. Biomolecular-based computation coupled with metamaterial modeling will inform all the design aspects of the system from molecular, and meta-atom to systems level scales. Random access in such systems will be achieved dynamically via deep subwavelength electromagnetic modulation, providing high capacity, light speed readout capability that breaks the limits in transient electronics. This project is supported by a $1.5M NSF SemiSynBio III grant. |
Rizal Hariadi | Experimental | Biological and Soft Matter Physics, Nanoscience and Materials Physics | Chiral Metamaterials for Information Storage | Big data has increased the demand for technological innovations in data storage while traditional electronics are reaching their physical limit. DNA is an emerging data storage material as a viable alternative to silicon-based technology due to its high capacity and low operational energy. However, current DNA storage technologies lack the structural access and allocated organization of data encoded in DNA, and the core operations require complex and slow writing and readout processes such as sequencing, which leads to practical barriers in speed, sustainability, and scalability. To address these challenges, this project aims to develop the first DNA-encoded metamaterial data storage system, which could lead to a rewritable and high-speed integrated random access. Uniquely integrating DNA nanotechnology with an optical metamaterial platform, information bits will be designed and encoded by the helicity or handedness with DNA origami-enabled chiral meta-atoms. Biomolecular-based computation coupled with metamaterial modeling will inform all the design aspects of the system from molecular, and meta-atom to systems level scales. Random access in such systems will be achieved dynamically via deep subwavelength electromagnetic modulation, providing high capacity, light speed readout capability that breaks the limits in transient electronics. This project is supported by a $1.5M NSF SemiSynBio III grant. |
Rizal Hariadi | Experimental | Biological and Soft Matter Physics, Nanoscience and Materials Physics | Structures and functions of molecular machines under mechanical tension | We aim to investigate the cell membrane force receptor of single-molecule integrin signaling pathways, dynamics, ligand-binding kinetics, and structure in the presence of realistic applied multiaxial tension enabled by DNA origami multiaxial tension device that we propose combined with single-molecule fluorescence resonance energy transfer (FRET), fluorescence correlation spectroscopy (FCS) and Cryo-Electron Microscopy (CryoEM). We will study two different types of integrins, Alpha1-Beta1, and Alpha5-Beta1, strongly related to the cardiovascular system and diseases, especially in cardiac fibrosis, as well as during healing and remodeling after a heart attack. If successful, the study will contribute to a better understanding of Alpha1-Beta1 and Alpha5-Beta1 integrins signaling pathways and structure under tension, leading to a more complete view of cardiac fibrosis and a therapeutic strategy for cardiovascular diseases targeting integrins. |
Rizal Hariadi | Experimental | Biological and Soft Matter Physics, Nanoscience and Materials Physics | Structures and functions of molecular machines under mechanical tension | We aim to investigate the cell membrane force receptor of single-molecule integrin signaling pathways, dynamics, ligand-binding kinetics, and structure in the presence of realistic applied multiaxial tension enabled by DNA origami multiaxial tension device that we propose combined with single-molecule fluorescence resonance energy transfer (FRET), fluorescence correlation spectroscopy (FCS) and Cryo-Electron Microscopy (CryoEM). We will study two different types of integrins, Alpha1-Beta1, and Alpha5-Beta1, strongly related to the cardiovascular system and diseases, especially in cardiac fibrosis, as well as during healing and remodeling after a heart attack. If successful, the study will contribute to a better understanding of Alpha1-Beta1 and Alpha5-Beta1 integrins signaling pathways and structure under tension, leading to a more complete view of cardiac fibrosis and a therapeutic strategy for cardiovascular diseases targeting integrins. |
Rizal Hariadi | Theoretical | Biological and Soft Matter Physics, Nanoscience and Materials Physics | DNA origami nanoarrays for studying biophysics | This project, supported by the prestigious National Cancer Institute IMAT R61 program, is developing membrane-spanning molecular machines for non-invasive detection of exosomal microRNAs. At its core, the project harnesses advanced principles of membrane physics and optical fluorescence to create a lysis-free, isothermal amplification-enabled sensing platform. Ph.D. students engaged in this project will be helping in the design and characterization of different types of nanoarrays, such as DNA origami nanoarrays and zero-mode waveguides for digital assays of exosomes. Their work is pivotal in pioneering digital assays of exosomes, thereby bridging the gap between theoretical physics and practical biomedical applications, and pushing the boundaries of how physical principles can be applied to advance medical diagnostics. |
Rizal Hariadi | Theoretical | Biological and Soft Matter Physics, Nanoscience and Materials Physics | DNA origami nanoarrays for studying biophysics | This project, supported by the prestigious National Cancer Institute IMAT R61 program, is developing membrane-spanning molecular machines for non-invasive detection of exosomal microRNAs. At its core, the project harnesses advanced principles of membrane physics and optical fluorescence to create a lysis-free, isothermal amplification-enabled sensing platform. Ph.D. students engaged in this project will be helping in the design and characterization of different types of nanoarrays, such as DNA origami nanoarrays and zero-mode waveguides for digital assays of exosomes. Their work is pivotal in pioneering digital assays of exosomes, thereby bridging the gap between theoretical physics and practical biomedical applications, and pushing the boundaries of how physical principles can be applied to advance medical diagnostics. |
Robert Kaindl | Experimental | Nanoscience and Materials Physics | Broadband Ultrafast Probes of Quantum Materials | Our research explores fundamental and applied physical phenomena in correlated and nanoscale quantum materials using advanced ultrafast tools. Femtosecond light pulses provide unique opportunities to perturbatively resolve fast dynamics and interactions in these materials, and they can drive materials into new transient or metastable regimes via intense excitation. We are developing a new laboratory for tailored driving and detection of quantum and collective excitations using broadband terahertz, mid-IR, and visible light pulses as well as probing of electronic structure dynamics via time- and angle-resolved photoelectron spectroscopy (trARPES). This effort provides many project opportunities, spanning initially the range from literature research and simulations, fabrication and characterization of 2D and correlated samples, to the design and development of nonlinear optical setups in the terahertz, mid-IR, and extreme-UV regimes. Moreover, our research is closely aligned with the ASU CXFEL program making possible laser-science projects connected to the femtosecond X-ray source and pump-probe setup that is currently under commissioning. At a later stage, the student can also get involved with the first time-resolved THz/mid-IR and ARPES experiments in our group to investigate the dynamics of quasi-particles and order parameters as well the properties of light-induced phases in two-dimensional, topological, and superconducting materials. |
Robert Nemanich | Experimental | Nanoscience and Materials Physics | Interfaces of Ultra Wide Bandgap Semiconductors and Dielectrics | Ultra wide bandgap semiconductors are now projected to be crucial for the future electricity grid, which will combine renewable energy sources, battery storage, bidirectional power distribution and DC and AC networks and transmission. High voltage electronics based on ultra wide bandgap materials such as diamond, boron nitride and aluminum nitride are central to achieving this goal. This research rotation project will employ photoemission spectroscopy to measure interface properties of wide bandgap oxide layers on doped diamond. The results will be important for design and fabrication of a new generation of power transistors. |
Robert Ros | Experimental | Biological and Soft Matter Physics | Exploring Biomolecular Dielectrophoresis with Nanoprobes | Dielectrophoresis (DEP) refers to the migration of polarizable particles in an electric field gradient. DEP has been successfully applied to bioanalytical applications for bioparticles such as bacteria and organelles, whereas established theoretical frameworks exist for such settings. However, the foundations of biomolecular DEP are still not fully revealed. An experimental challenge still remains for assessing the forces biomolecules are subjected to in dielectrophoretic studies, which would on the other hand allow to uncover the fundamental biomolecular properties leading to their frequency dependent polarization and the underlying theoretical framework. This project will address this challenge by subjecting selected proteins, DNA, and RNA to dielectrophoresis using an atomic force microscope probe as nanoscale electrode in combination with fluorescence microscopy and spectroscopy. The student will develop the adequate experimental setup to characterize dielectrophoretic trapping forces of biomolecules in a range of conditions. Beside the determination of fundamental biomolecular properties, this work will be relevant for biomedical studies with the aim to gather important parameters to eventually develop diagnostic applications through biomolecular dielectrophoresis. The project will be carried out jointly in the biophysics laboratories of Dr. Robert Ros and the Biodesign Institute in Dr. Alexandra Ros’ laboratory. |
Rogier Windhorst | Experimental | Cosmology, Particle, and Astrophysics | Cosmology and Astrophysics Research with the Hubble and James Webb Space Telescopes | The student will study current hot topics in cosmology, the epoch of cosmic reionization, star-formation in a cosmological context, galaxy formation and evolution, gravitational lensing via galaxy clusters and cluster caustic transits, and the growth of super-massive black holes in the centers of galaxies. The student will will get hands-on experience and learn how to reduce and analyze Hubble and JWST data. We meet with the whole research group once a week (currently Fr. 1:30-3:30 pm in GWC-505) to assign projects, train students, monitor progress, and discuss specific research aspects, skills, and progress on papers. |