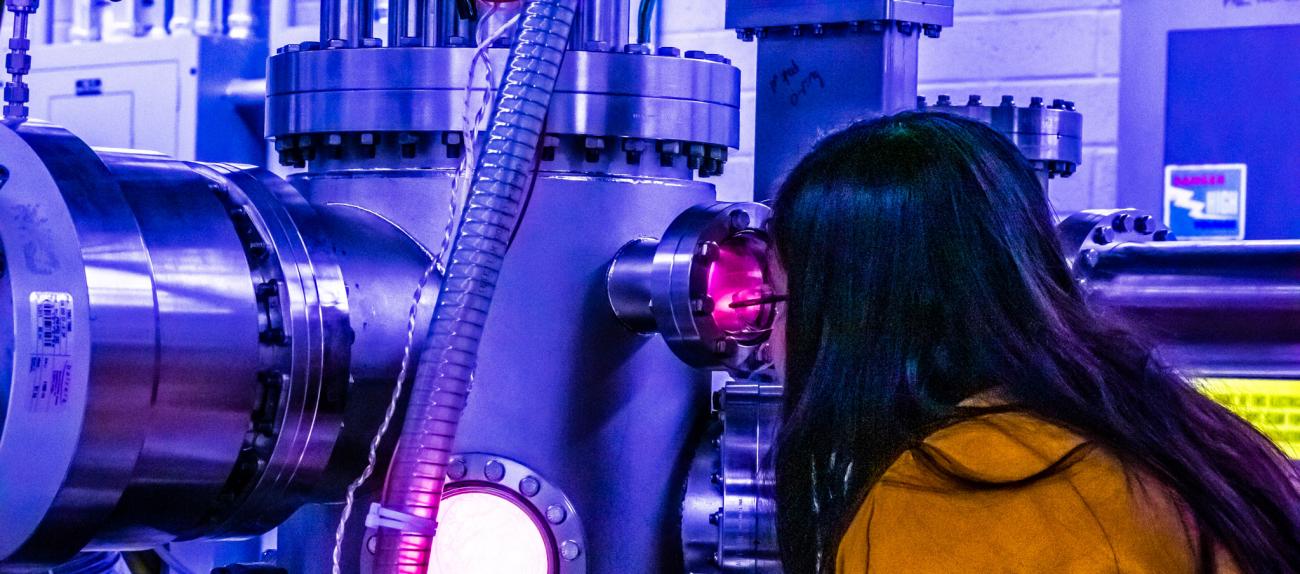
Undergraduate Research
Apply what you have learned in the classroom to real world problems. Not sure where to start? Take a look at the resources below.
Get started by finding an area of interest
Department of Physics Research Areas
The Department of Physics conducts research in four main focus areas of physics: biological and soft matter physics, cosmology, particle, and astrophysics, nanoscale and materials physics, and physics and society.
Check out our faculty's research
Faculty by research area:
Biophysics and Biological Physics, Cosmology, Particle and Astrophysics, Nanoscience and Materials Physics , Physics and Society
Faculty by initiative:
Biodesign Institute, Center for Biological Physics, Cosmology Initiative, Interplanetary Initiative
Apply to an opportunity
Research openings
Department of Physics faculty and affiliated faculty will periodically post openings in their group. Use the link below to view the current list of research projects.
Start the conversation
Interested in working with a physics faculty member? Send them an email to let them know you're interested in their research. Include the following in your email: relevant skills, grades of recent math and physics courses or unofficial transcript, and why you are interested in their group.
ASU Programs
Prefer a bit more structure? Apply to a program:
- LEAP Scholars (transfer)
- SCENE (high school)
- QRLSSP (math REU)
- Online Undergraduate Research Scholars - (Online)
Research Experiences for Undergraduates (REU)
The National Science Foundation funds research opportunities through its Research Experiences for Undergraduates (REU) Sites program. Participating in a REU is a great way to gain research experience and build lasting connections.
You've found a research opportunity, now what?
Gain academic credit
Physics majors can enroll in PHY 495 Project Research to obtain credit for conducting research with a professor in the Department of Physics. Students must secure a research instructor before registering.
Apply for funding
Here are a few websites where undergraduates can apply for research scholarships or grants:
Showcase your research
Present your research at the Department of Physics Annual Undergraduate Research Symposium or at a physics conference.